
Predicting Stress – Physiologically Measuring and Predicting Noise Effects
Abridged version of the publication in Lärmbekämpfung (Laufs, C., Herweg, A.: "Beurteilung von Lärmwirkungen anhand physiologischer Messungen", published in Zeitschrift für Lärmbekämpfung 18 /2022 Nr. 1, S. 11-16; VDI Fachmedien, Düsseldorf.) https://www.ingenieur.de/fachmedien/laermbekaempfung/
The "fight-or-flight response" has helped people cope with dangerous situations by fighting back or fleeing since time immemorial. It enables the body to adapt to such conditions – for example, by increasing the heart rate, which prepares it for physical exertion. In confrontations with wild animals or natural forces, such physical reactions ensured survival. Today, these factors no longer pose a threat to most people. Nevertheless, our brain continuously evaluates our sensory perceptions for indicators of potential danger. Sounds, in particular, can put our bodies on alert. Instead of wild animals, sounds and noise are primary stress triggers in modern society – along with factors such as time pressure or climatic conditions.
In the following, we provide an overview of how HEAD acoustics uses physiological measurements in listening tests to assess noise effects. In doing so, we describe the potential of physiological measurements in studying noise effects and how they can be used to predict stress effects.
Extra-aural noise effects
Noise can harm people and poses a potential health hazard. This is not just a matter of excessive sound pressure levels. The direct damage it causes to the auditory system is referred to as aural noise effects. Noise effects, however, are much more complex and go far beyond aural effects. [1] Noise effects that affect other parts of the body are called extra-aural noise effects. These include physiological or psychological stress and a weakening of cognitive performance – i.e., effects that only occur in the brain due to further processing of the sound events. Such extra-aural reactions to noise can have serious health consequences [2]. How does such a stress reaction unfold?
The physiology of a stress response
The body perceives certain sound events as indicators of potential danger and puts itself on the alert as a precaution by activating the sympathetic nervous system. Together with the parasympathetic nervous system, the sympathetic nervous system forms the autonomic nervous system, whose functions are mainly unconscious. In contrast to the sympathetic nervous system, the parasympathetic nervous system has a calming or dampening effect on the organism. If a stress trigger activates the sympathetic nervous system, the body releases adrenaline, leading to, among other things, an increased heart and/or respiratory rate. Muscle tone and blood pressure increase, and digestion shuts down to provide the body's energy for the crucial functions of fight or flight. Dilated pupils contribute to better vision. If such alertness occurs frequently, it can lead to long-term damage to health. Repeatedly elevated blood pressure or increased heart rate can thus lead to damage to the cardiovascular system, among other things [3]. If the body is under the persistent influence of a stressor, it even enters a so-called state of loss of control. If neither fight nor flight is possible or practical, the body adjusts to endure the stressor and releases cortisol after its prolonged influence. This stress hormone then leads, for example, to an attenuation of the pain response. However, the frequent release of cortisol can weaken the immune system long-term. The same applies to the stress reaction: the dosage makes the poison.
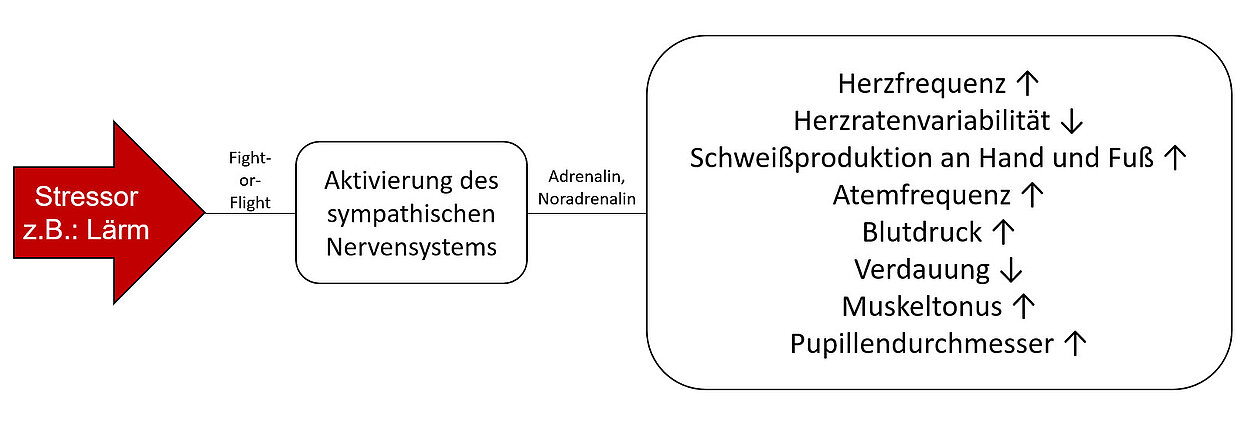
Fig. 1: Overview of the physical changes during a stress reaction
The good thing is that we can detect these stress reactions with sensors. The data collected enables the scientists at HEAD acoustics to assign extra-aural noise effects to specific sound events and go beyond simply questioning test subjects when investigating noise effects. And that is important because people do not always consciously perceive stress, even when measurable stress reactions occur in the body [4].
The physiological parameters
The most commonly used physiological parameters that common measurements capture include heart rate, heart rate variability (HRV), and skin conductance. The breathing rate, finger pulse amplitude, or brain activity can also be recorded.
In connection with acoustics, physiological measurements were already used in 1962. One of the first investigations of sounds with physiological measurements could establish a correlation between sound bandwidth and the finger pulse amplitude already at that time [5]. However, physiological measurements in acoustics require a large number of preliminary considerations to be successfully applied. Classical listening experiments, in which subjects participating in the investigation evaluate many sounds in rapid succession, no longer play a role. Instead, the subjects listen to sounds over a specific duration, and they are allowed and required to take breaks between stimuli. This is because stress reactions often not only result in a short-term change in certain physiological parameters, but it also takes time for these parameters to return to a resting value.
Artifacts
Artifacts, i.e., disturbances in the signal, pose another problem. They are mainly caused by movements of the test persons and make a valid evaluation of the physical response difficult. Measurements of heart rate by ECG or respiratory rate by chest strap are less susceptible to motion artifacts than skin conductance measurements.
On the other hand, when measuring skin conductivity, movements change the contact of the electrodes and thus also the measured conductivity. Studies in which the test subject has to move the hand with the electrodes, for example – including studies in vehicles – therefore present a particular challenge. Skin conductivity measurements on the foot soles or combined measurements with electrodes on the hand and foot can provide better data quality in such cases. Deep breathing and even speaking can also lead to increased sweat production and thus different skin conductance, resulting in increases in the signal similar to stress-induced increases in skin conductance. Therefore, listening tests should do without verbal feedback during the measurement and instead request responses via the mouse and keyboard. An additional recording of breathing may indicate such rises caused only by a very deep breath. Other options for artifact correction via corresponding algorithms are available for all measurement types.
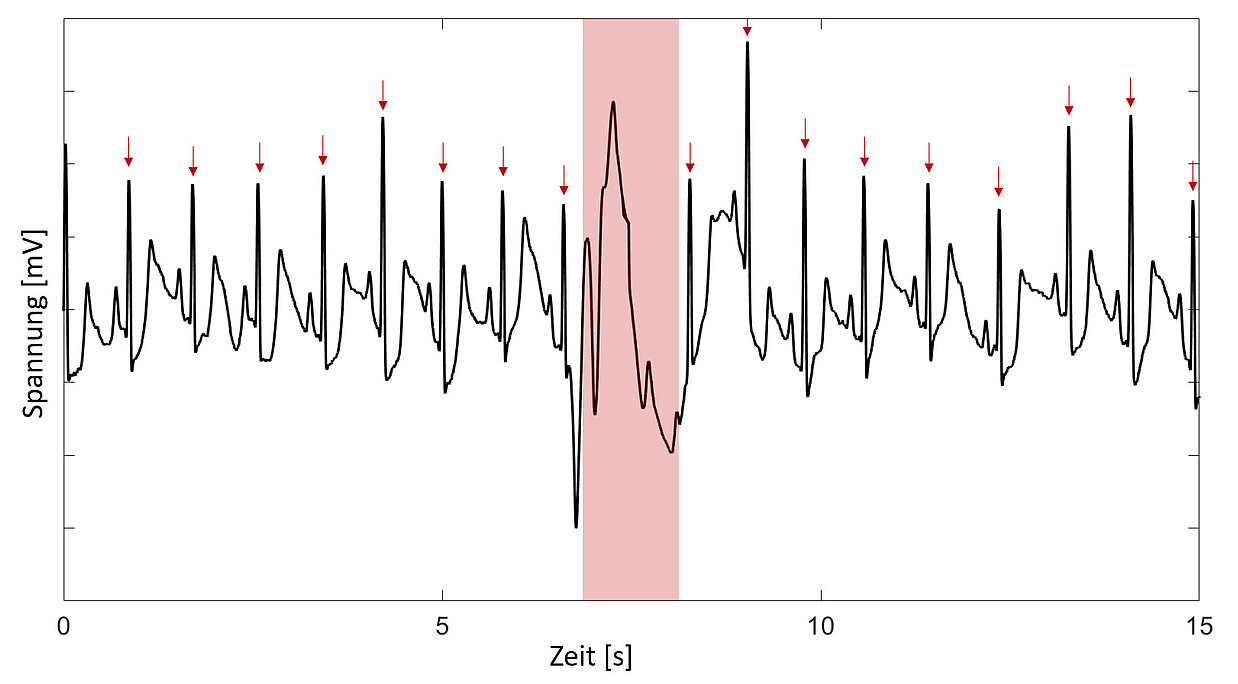
Fig.2: Artifacts in the EDA signal
Stress response and psychoacoustics
Studies with physiological measurements involve considerable effort – even if a set of psychoacoustic parameters already exists that reflects how listeners feel about sounds. These parameters make a valuable contribution to the qualitative description of noise effects. For example, the DIN ISO 12913 soundscape standard [6] suggests that it is not enough to make unwanted sounds quieter. Instead, we use psychoacoustic findings to evaluate the overall acoustic situation more comprehensively and, thus, better. For example, surveys of test subjects have revealed correlations between the sharpness and the annoyance of a sound.
Our research does not investigate the physiological response to a specific sound but to the psychoacoustic properties of sounds to determine their connection. Conclusive results would allow us to predict part of the stress effect of a sound via psychoacoustics without the need for elaborate physiological measurements each time.
The relationship between sharpness and stress response
First, we investigated the relationship between the sharpness of a sound, i.e., the high-frequency components of the total loudness, and the stress response. Since higher sharpness is often associated with higher rated annoyance, one might expect a more robust stress response to accompany it. Therefore, we used noise of different sharpness as stimuli – all other noise parameters did not change. A cognitive test integrated into the experimental procedure prevented long periods of inactivity, which could make valid conclusions difficult. In this test, all subjects performed the same controlled cognitive activity that more realistically represents a typical work environment than inactivity. Such a cognitive test additionally offers the possibility to investigate the influence of noise on cognitive performance – another extra-aural noise effect.
We recorded and analyzed the subjects' skin conductance to assess the stress response. Observation of respiration and algorithms to correct artifacts before analysis increase signal quality.
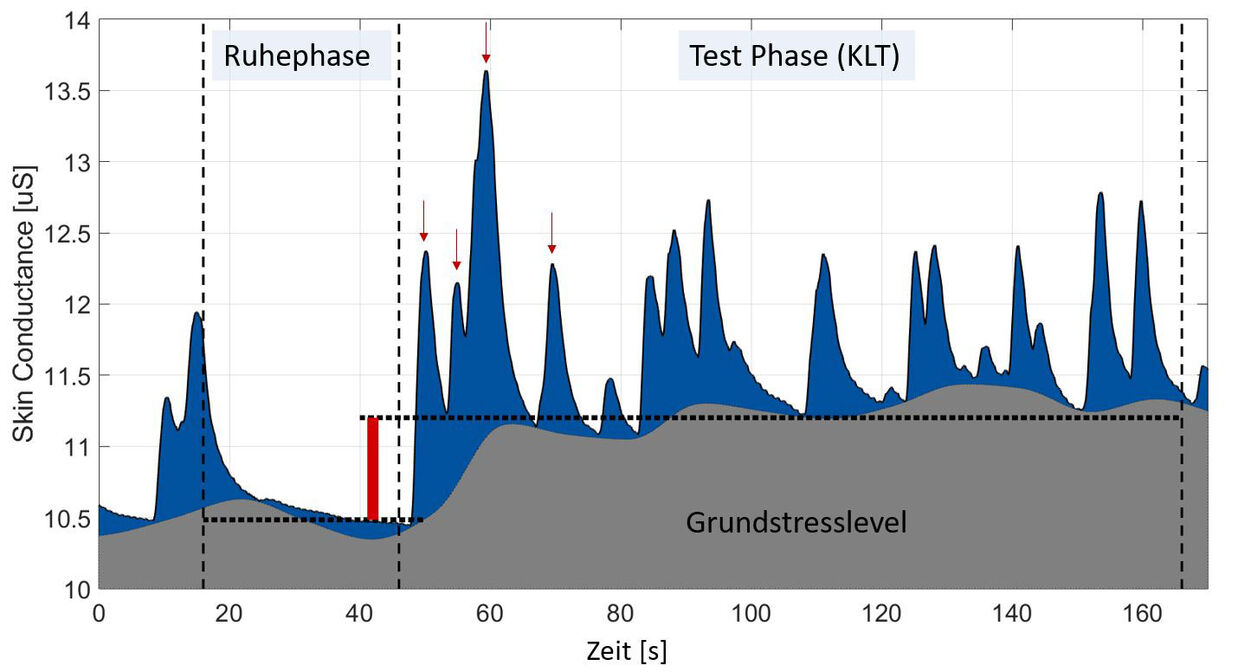
Fig. 3: Signal at rest and testing
The data analysis showed the expected more robust stress response with the higher acuity sound. It was statistically significantly higher than the response to the low acuity noise and activated the sympathetic nervous system more strongly. Therefore, we can assume that noise of higher sharpness has a greater potential to cause adverse health effects.
The results of the cognitive performance evaluation look different. In contrast to what we had previously assumed, the subjects achieved significantly better results for the noise with higher sharpness. According to the capacity-resource approach [7], stress depletes attentional resources, reducing the use of task-irrelevant processes in the brain. The human body enters the fight-or-flight response and requires brain resources to assess the stressor and control it. However, related to this study experiment, an increased stress response due to the higher sharpness of the noise possibly leads to a higher concentration on the processing of the arithmetic tasks. Thus, concerning cognitive performance, we can speak here of positive stress (eustress). Nevertheless, the sympathetic nervous system has more robust activation, which can have long-term health consequences.
Future research
In addition to skin conductivity, we are also investigating heart rate variability and brain activity in initial preliminary studies at HEAD acoustics. In the future, these should enable an even more comprehensive evaluation of the physiological response to sounds. With a new experimental design for the investigation of noise, we are thus investigating the relationship between additional psychoacoustic parameters and stress response.
Further improvements in the avoidance and removal of artifacts already allowed first analyses of the physiological response in more active scenarios, such as the driver's reactions while driving a vehicle. In this way, we can relate the physiological responses to specific driving situations or compare different vehicles.
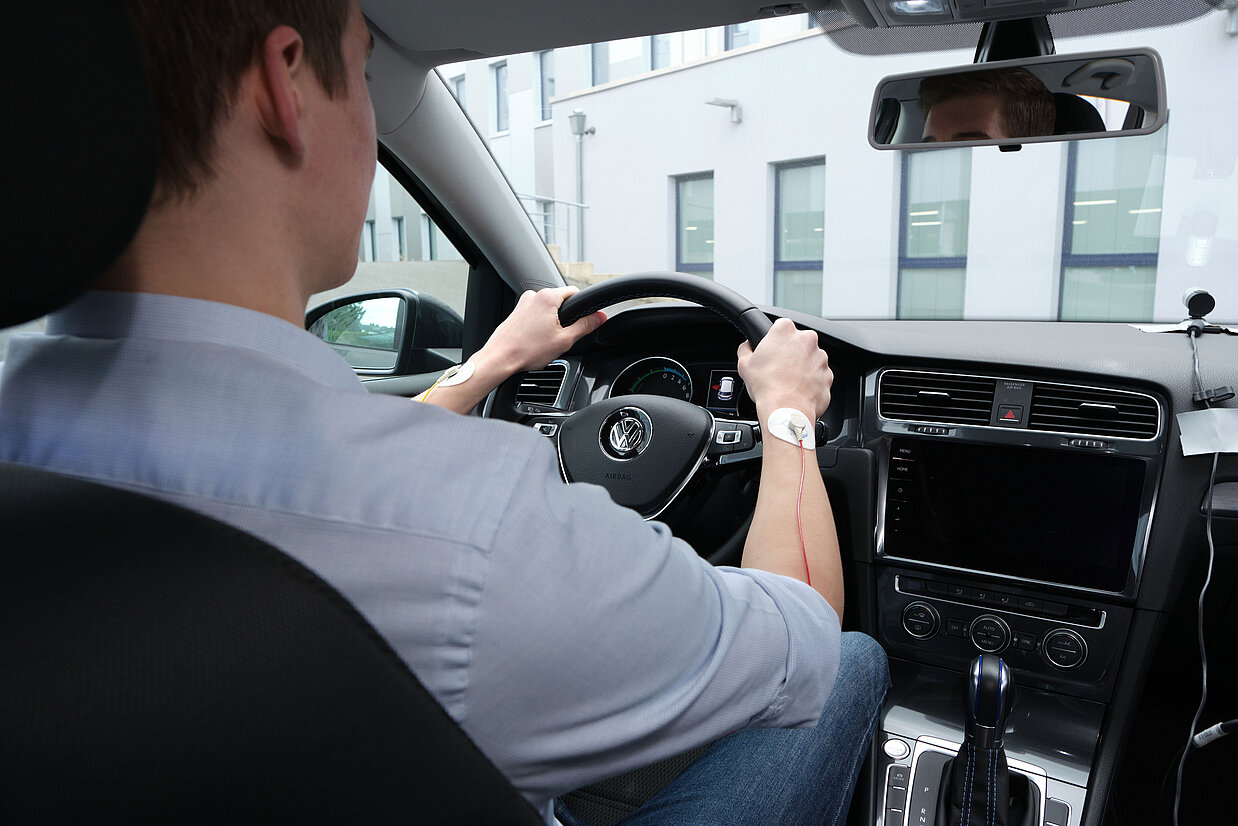
Fig. 4: Sensors in the car
Summary and conclusion
The potential of physiological measurements in assessing noise effects is considerable, but further research is needed to exploit this potential. With interference-free signals and a valid measurement methodology, which in turn allows reliable statements about the physical situation, we have already taken a big step. Together with a hearing test design optimized for the investigation of noise and the appropriate analysis of several simultaneously recorded physiological parameters, we can exploit this potential. Thus, in the future, we could directly predict whether a sound could elicit a stress response and, ideally, avoid it.
[1] E. Daniel, „Noise and hearing loss: a review,“ Journal of School Health 77.5, p. 225–231, 2007.
[2] H. Faller und H. Lang, Medizinische Psychologie und Soziologie, 2006.
[4] M. Spreng, „Central nervous system activation by noise,“ Noise and health, p. 49, 2000.
[5] G. Jansen und P. Y. Rey, „Der Einfluß der Bandbreite eines Geräusches auf die Stärke vegetativer Reaktionen,“ Internationale Zeitschrift für angewandte Physiologie einschließlich Arbeitsphysiologie, pp. 209-217, 1962.
[6] „DIN ISO/TS 12913-3:2021-06; Akustik - Soundscape - Teil 3: Datenanalyse (ISO/TS 12913-3:2019)“.
[7] E. Chajut und D. Algom, „Selective attention improves under stress: implications for theories of social cognition.,“ Journal of personality and social psychology, p. 231, 2003.
Find the full article (in German) in the magazine "Lärmbekämpfung": doi.org/10.37544/1863-4672-2022-01-13